The Oil & Gas Industry has faced turbulent times due to oil prices, which decreased from a level higher than $100.00 to its lowest price in the last two years, reaching $28.50 in January of this year [1]. In this macroeconomic context, great efforts have been employed by major operators in the direction of significant cuts in cost and reduction of development activity for new projects.
For the projects that remained active, there have been a growing demand for increased efficiency in economic performance, with focus on minimizing CAPEX. With this perspective, the concept selection phase is presented as a key step in the process, when it is possible to achieve significant cost reductions through the systematic evaluation of a large population of alternatives, covering the largest amount of conceptual architectures as possible.
The usual methodology adopted in the development of conceptual projects is based on a multi-disciplinary approach, in which the work packages associated to each discipline are developed sequentially, and each discipline determines the technical requirements and boundary conditions for the next discipline. Based on this workflow, the financial evaluation of the conceptual alternatives occurs after the technical definitions, which makes it difficult in many cases the execution of optimization processes with focus on economic indicators, since the schedules are usually limited to accommodate several cycles of project tuning in a non-integrated way. Other practices associated with the usual philosophy of project also have significant influence on the results achieved in each development. Aspects such as the organizational structure, the low tolerance of companies to technological risks, the specialization of the regional supply chain, the accuracy of the engineering methods and practices related to the cultures and paradigms observed in different Oil & Gas production poles are relevant points of influence in the overall project efficiency.
This paper aims to present a management review of the critical aspects associated with the offshore full field developments, with emphasis on technical, economic and organizational practices associated with the usual project methodology employed by most of companies in the sector.
OFFSHORE OIL & GAS PRODUCTION SYSTEMS
With the depletion of the onshore oil fields, the Oil & Gas Industry has expanded its operations to the sea, in water depths increasingly higher. In addition to the difficulties of finding oil in remote regions of the globe, the development of offshore production fields at large distances from the coast and at high depths presents several technical and economic challenges. In general, the development of an offshore production field can be considered as a multidisciplinary development, involving highly trained engineers in various technical disciplines, together with a project management team that has all capabilities to assess the economy of the investment and manage its development, while maintaining strict controls on cost, time and quality at the various stages.
In a technical perspective, the development of an offshore production field follows a process technically oriented in which each discipline determines the technical requirements and boundary conditions for the next discipline. The normal sequence starts in the reservoir engineering, followed by wells engineering, subsea engineering, flow assurance, topside facilities and production unit. The methodology for the development of the conceptual design may vary between companies on the logical sequence, on accuracy of each phase and in scope, but in general, there is a strong influence of the organizational culture and of the supply chain in the target region [2].
In an attempt to reduce the uncertainties about the economic viability of a venture in the order of billions of dollars, it is invested a lot of time and financial efforts in the phase of prospection and exploration, where data on the reservoir is collected through seismic surveys and through exploratory wells. The exploration phase comprises all activities and studies necessary to detect and measure the occurrence of hydrocarbons. It is commonly used the seismic studies and data from previous explorations campaigns to find oil. Once the occurrence of hydrocarbons is observed, the Company proceeds with the “appraisal” phase, which consists on a drilling campaign carried out with the objective of assessing the extent of the field, the reserves, the potential productivity, and the properties of the oil or gas.
In addition to the reservoir engineering, other technical disciplines – such as wells engineering, subsea engineering, topside facilities and production unit – demand a highly significant investment in the project execution phase, and are usually the bottlenecks in terms of schedule of development, and often in technological terms to deal with projects technically challenging. In its turn, the flow assurance analyses integrates all technical disciplines, reflecting the production capacity of the reservoir into technical requirements for equipment and systems of the other technical disciplines.
PROJECT PHASES AND ACCURACY IN COSTS
Given the magnitude of investment observed in an offshore full field development, it is necessary to adopt appropriate methods and processes to ensure the economic viability of the projects, as well as to effectively control the fulfillment of scope, schedule, quality and risk mitigation. In the last decades, various initiatives have been observed to organize the knowledge about project management, with emphasis on the Project Management Institute [3] and on the Project Independent Analysis [4].
The Oil & Gas Industry has consistently used the combination of both methodologies of PMI and IPA in the development of major projects, with particular attention to the methodology FEL, which combines an approach of “Rolling Wave Planning” [5], with a vision of technical and cost integration in the light of empirical tools of IPA [4]. Figure 1 shows the typical phases of an offshore full field development project, adapted from BARBOSA et al. [6].
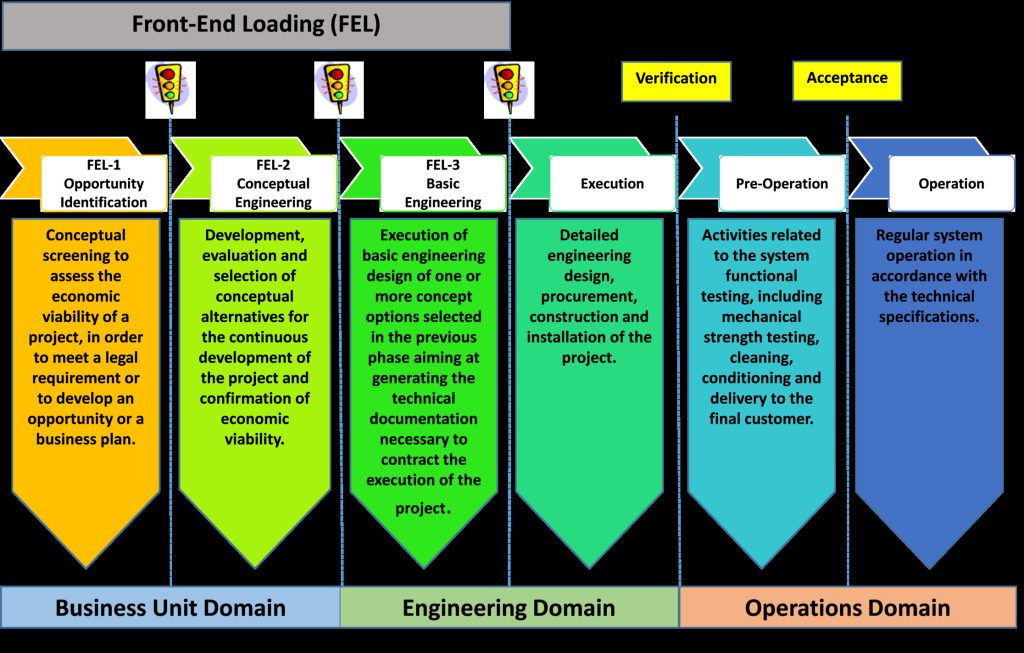
As shown in Figure 1, it is observed that the FEL methodology is focused in the early stages of project, aiming at increasing progressively the level of maturity of technical information, limiting investment in each phase, and ensuring that the decision-making about the continuity of the project in each phase can be developed based on both technical and financial documentation. After the approval of the FEL 3, it is understood that the level of maturity is appropriate for the execution (construction) of the project.
FEL 1: Opportunity Identification: This is the business assessment phase, when it is verified the strategic alignment with business plan and market opportunities. The engineering associated with this phase is based on index of similar projects. This step involves the definition of scope and objectives of the project, as well as an initial estimate of the amount of investments required, by providing a range of variation in the cost, in the best estimate, between -20% and +30%, according to Class 5 of the “cost estimates classification matrix”, defined by AACE International [7]. At this stage, it is also performed the calculation of the main viability indicators, such as IRR, NPV and Discounted Payback.
FEL 2: Conceptual Engineering: This is the stage of development, evaluation and selection of conceptual alternatives. The main focus of this phase is the development of the conceptual engineering of all options listed in the FEL1, in order to compare the options and define, through the results of the financial-economic assessment of each option, which alternative will be developed in the next phase. At this stage, it is performed analyses of technological and construction solutions available, aiming at evaluating and selecting the concept alternative, along with the development of conceptual specifications and cost estimates, within a range of variation, in the best estimates, between -15% and +20% if considered as a reference Class 4, or between -10% and +10% if considered as a reference Class 3, as defined by AACE International [7]. At this stage, it is usually developed workshops of VIP’s (Value Improving Practices) to guide the basic engineering phase. It is possible that more than one conceptual alternative be recommended for the next stage. This option has been observed in projects of high technological complexity, when a design competition may be recommended in the next phase. In FEL 2, the financial kpi`s are crucial to approve the project to proceed to the third phase: if the return on investments are not above the minimum rate of attractiveness, i.e., calculated NPV is less than zero, the project should be canceled or revised.
FEL 3: Basic Engineering: In this phase, the focus is the construction, i.e., the preparation of the project for its corporate approval and future implementation. At this stage, the basic engineering of the selected option in FEL 2 is performed, allowing the calculation of CAPEX of the project with greater precision. The engineering solution selected in FEL 2 is technically detailed and more VIP’s are considered in the development of the basic engineering design. In this way, it is possible to obtain a variation in costs, in the best estimate, between -5% and +5%, according to Class 2 of the “cost estimates classification matrix”, defined by AACE International [7], in addition to the consolidation of the main kpi`s of economic viability.
The correlation of the classification of cost estimate defined by AACE International [7] and the methodology FEL [6] follows specific business criteria defined by each Company. In some Corporations, the FEL phases are subdivided, generating sub-phases, as FEL 2a and FEL 2b. This subdivision reflects the need to create intermediate gates for decision-making, seeking to prevent that long stages will lead to inadequate solutions regarding the achievement of general interests of the Company, in spite of having produced satisfactory kpi`s. Figure 2 shows the accuracy ranges in the cost estimates based on the classes, represented by the blue area of the chart, according to AACE International [7].
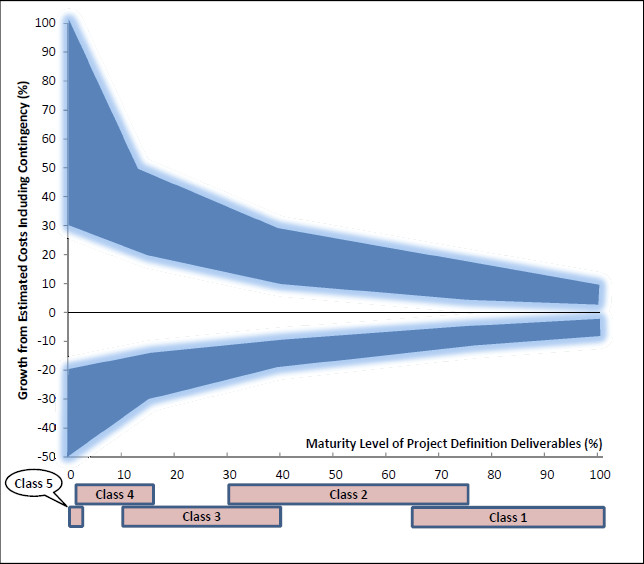
Based on the description of the phases of an offshore full field development project, it is possible to conclude that the level of integration between the various disciplines in the phases of FEL 1 and FEL 2 is crucial in the generation of optimized solutions with focus on financial and economic kpi`s. Due to many limitations and constraints observed in an offshore project for the Oil & Gas Industry, the generation of alternatives in these phases has been usually based on the development of concepts with the greatest technological maturity and with the lowest CAPEX as possible. Based on this usual approach, the generation of optimized concept options, with focus on NPV, presents a high cost in terms of time and required engineering resources. Therefore, the evaluation of the NPV of each conceptual alternative is usually performed as an isolated function of the finance discipline, calculated at the end of the engineering process.
CONCEPTUAL PHASE GENERAL PROCESS
Based on the practices observed in the global offshore poles, it is possible to notice that the environmental and historical conditions, and especially the culture of organizations operating in each region, define the practices and technological trends. On the other hand, there is a great similarity between the various regions regarding the logical sequence in the technical definition of concepts, in which the work packages associated to each discipline are developed sequentially, and each discipline determines the technical requirements and boundary conditions for the next discipline. Based on this workflow, the financial evaluation of the conceptual alternatives occurs after the technical definition phase, limiting in many cases the optimization process with focus on economic kpi`s, given the limited schedule usually available. Therefore, it is possible to conclude that the level of integration between the various disciplines is limited, since the technical processes have excessive focus on delivering reports and input data required to progress in the next discipline, and less efforts in developing integrated economic analysis of sensitivity for the various design variables. Figure 3 shows a typical workflow in the generation of conceptual alternatives in an offshore full field development.
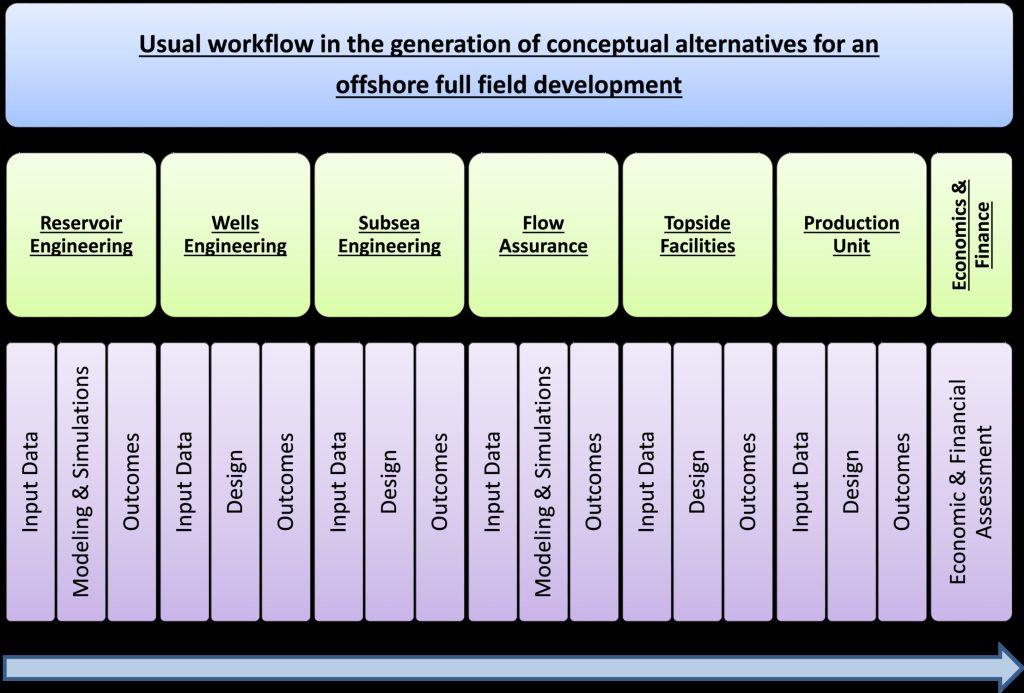
The workflow presented in Figure 3 usually requires several months to generate a limited amount of conceptual alternatives, since the organization of work is mostly sequential, and in certain disciplines, the engineering methods and processes are based on computational tools with an accuracy level much higher than what is required in the phases FEL-1 and FEL-2, despite the large uncertainties inherent to the data available in these phases. This practice leads to analyses that can reach several weeks of computational simulation for just a basic concept and long cycles of revision of the analyses carried out whenever a given input data is updated or changed.
ASPECTS RELATED TO SCHEDULE – FAST TRACK PROJECTS
In fast track projects, it is developed parallelism between the technical disciplines, particularly in the disciplines associated with design of equipment and physical systems – such as the well engineering, subsea engineering, topside facilities and production unit – in which the specification and acquisition of equipment and systems are usually in the critical path for the project development. Once the computer simulations of reservoir and flow assurance depend on the technical definitions of equipment and physical systems, it is common to verify reworks after equipment and systems specifications, leading to several cycles of revisions and design changes that often consume the time savings with the fast track approach, and usually leads to higher costs than those observed in a regular project schedule.
ASPECTS RELATED TO MATRIX STRUCTURES
Another aspect observed in large corporations of the sector is the adoption of matrix organizational structure, oriented by technical disciplines. In the matrix structure, a project manager or a project coordinator is appointed to lead the integration and the development of the project along with a technical team, formed by members of various functional departments. The creation of a matrix structure is usual when there are several similar projects composing an investment portfolio, a situation in which the matrix structure may promote synergy between the various projects and create a favorable environment for the enhancement of specialization and exploitation of lessons learned among the projects. On the other hand, the management of the technical teams inside the functional departments is of a reasonable complexity, once the activities assignments is routinely performed by the project manager, without, in many cases, an accurate control of resources allocation between various projects. The result usually observed is the sharing of specialized professionals in various projects, hindering the prioritization of activities dedicated to the optimization of projects with economic focus. Another characteristic of the matrix structures is the limited level of integration between the technical teams, once the specialists are, in most cases, physically allocated in separate locations and should follow the guidelines and goals defined by their own departments. Therefore, the communication and integration between the technical disciplines tend to be jeopardized.
ASPECTS RELATED TO TECHNOLOGICAL RISKS
In addition to aspects associated with interdisciplinary and organizational issues, it is usually observed in most of companies in the sector a low level of tolerance to risks of technological maturity of equipment and innovative systems. Despite the literature and standards available to guide processes of development and qualification of new equipment and systems in the Oil & Gas Industry, what is observed in practice is a tendency to the selection of qualified equipment and systems, field tested and in operation in other assets (field proven), or even already used by other companies. This approach usually leads to long cycles of maturation of new technologies, despite the benefits observed with the innovation. In scenarios determined by high demand and high prices of the commodity, the tolerance to technological risk is further reduced, since there is no need for great efforts of innovation to achieve the economic feasibility of projects with favorable rates of return.
ASPECTS RELATED TO SUPPLY CHAIN
In its turn, the regional supply chain have demonstrated a significant influence in the decision-making process about which design archetypes will be adopted in the development of conceptual alternatives. Based on the historical aspects of each offshore production pole, it is observed a specialization of the regional supply chain to provide certain types of equipment and systems. Due to geographical, politics, fiscal aspects and also local content constraints, the full globalization of the supply chain becomes unattractive and in many cases uneconomical, feeding back the specialization cycle of the local supply chain to provide specific equipment and systems. Because of this specialization in the regional supply chain, most of Companies has become less prone to effectively adopt different design archetypes, despite the positive margin verified in the economic evaluations of concept designs different from the ones historically selected. The logistical difficulties of importation of equipment, and often the lack of local availability of special naval resources – necessary for the construction of the offshore system – leads to additional logistical risks that also influence the decision makers.
ASPECTS RELATED TO THE ACURACY OF THE ENGINEERING METHODS
Between the aspects already discussed, the excessive accuracy of engineering methods applied to the project feasibility assessment (FEL-1) and for the selection of conceptual alternatives (FEL-2) may lead to a significant impact on final economic results. Once refined computational methods requires more time to perform the analyses and also a higher level of precision for the input data, it is not uncommon to observe a large number of revisions throughout the process due to updating’s of environmental and reservoir data, which are usually of low reliability at the conceptual phase. This practice makes unfeasible to evaluate a larger number of alternatives, since the search for an excessive accuracy leads to few conceptual options fully evaluated within the timeframe available. According to the guidelines defined by the AACE International [7], the accuracy of cost estimates for Class 5 shall be, in the best estimate, between -20% and +30%. In fact, as presented in Figure 2, a cost estimate for a feasibility analysis tolerate an accuracy range between -50% and +100% in Class 5 and between -30% and +50% in Class 4. This observation inevitably leads to a reflection about the accuracy of the engineering methods adopted on the phases FEL-1 and FEL-2, in which analytical processes and empirical correlations are fully accurate to deliver the required accuracy, and with computational costs significantly lower. An example of this approach is presented by BEHNIA [8], in which the accuracy of the results of an empirical correlation applied to a multiphase flow presents an average error of 14% toward the conservative side. In other words, empirical formulations and analytical methods can deliver satisfactory results for FEL-1 and FEL-2, according to guidelines presented by AACE International [7].
CULTURAL ASPECTS OF ORGANIZATIONS
Despite all aspects previously discussed, each Company develops its own culture and practices applied to the development of offshore production system. Therefore, there is a strong tendency of repetition of methods and processes, and the use of equipment and systems already known, that brings comfort to all professionals involved. In more challenging scenarios as experienced at the time of writing this study, companies are led to test new methods and processes. However, the structural changes, necessary to allow new techniques to succeed, should pass through cultural changes throughout the entire organization.
TECHNOLOGY TENDENCIES IN THE WORLD SCENARIO
In the current economic context, formed by the combination of high costs of project development with low oil prices, the development of new technologies, methods and processes has been encouraged and led by major companies in the industry. Some tendencies and developments can be highlighted, such as subsea processing systems; intelligent wells technologies; new subsea concepts and equipment; and real time monitoring, data analysis and production management technologies.
The subsea processing is a solution adopted by Oil Companies to access the reserves located in remote areas or where the environmental conditions are severe, such as the North Sea, the Arctic and Alaska; and also in deep waters of Brazil and the Gulf of Mexico. The advantages of bringing the processing equipment to the seabed are to maximize and accelerate the recovery of oil, increasing its recovery factor and extending the life of field; and reducing the number of floating production units and surface processing plants [9].
According to information collected by industry experts, the discipline of wells engineering is responsible for about 40% of the development costs of a typical offshore project in ultra-deep water. In order to reduce the overall investment of the project, several initiatives have been undertaken in the search for greater efficiency in methods and processes of construction of subsea wells. In addition to the optimization of processes, innovative technologies such as smart completion systems allow multiple production zones to be produced by the same well, which reduces the need for investment in the exploration and production of an area consisting of several reservoirs or various production zones.
From the subsea engineering perspective, the development of concepts is an activity with endless possibilities and is strongly influenced by historical, political, environmental and cultural aspects of the supply chain of each region. Therefore, the development of lessons learned based on experience observed in other regions and the integration of the various disciplines have received great attention of top management team inside the major Corporations. On the other hand, the cultural aspects of each Company have been the main barrier toward the diversification and the development of conceptual options different from those already known and dominated by the corporation.
From the information technology perspective, the development of large databases, known as “big data”, has allowed industries of various segments to transform the way the automation has been applied, enabling real time monitoring and control of operations. In the Oil & Gas Industry, several initiatives have been observed in the development of technologies for real time monitoring and control of equipment and systems. Following a global tendency known as Industry 4.0, it is expected that this technological wave achieves fast the Oil & Gas Industry, supporting the real time management of offshore oil and gas fields, allowing a better production management and consequently leading to economic efficiency of assets. According to Howell [10], the “Oil and Gas Digital Field” is a tendency in the industry, and will make possible to maximize economic returns on investment facing the dynamism in the behavior of a petroleum reservoir.
CONCLUSIONS AND RECOMMENDATIONS
Based on the presented context, it is highly opportune the development of computational tools to allow the integrated generation and assessment of offshore concepts, within the accuracy range required for FEL-1 and FEL-2. The ranking of concepts through economic kpi`s such as internal rate of return (IRR) and net present value (NPV) leads to a greater objectivity in the process and reduces the subjectivity inherent to the different cultures and paradigms observed in each offshore production region.
Integrated models applied to concept generation should integrate the various disciplines simultaneously, including reservoir, wells, subsea, flow assurance, topside facilities, production unit and finance. This approach may bring significant performance in the concepts generation regarding to the processing speed and the number of options generated and evaluated.
In a scenario economically challenging for the Oil & Gas Industry, it is highly recommended that innovative technologies be considered in the development of offshore production systems. Based on the literature and standards available to guide process of development and qualification of new technologies in the Oil & Gas Industry, the risk associated with new technologies can be accordingly mitigated through an appropriate technology management plan developed and applied in the early stages of every project. Given the usual timeframe of a full field development, it is totally feasible to develop most of innovative technologies on time to be adopted in FEL-3, with significantly low cost efforts if compared to the economic benefits brought by such technologies.
Regarding to the accuracy level of the engineering methods, it is recommended to adopt in FEL-1 and FEL-2 computational tools more agile and aligned to the accuracy range required for the early stages of project, that is in the best estimate from -20% to +30% [7]. This practice promotes greater efficiency in the use of critical resources and a holistic evaluation of the most varied possibilities in terms of viable conceptual options and design archetypes.
REFERENCES
1. http://www.macrotrends.net/1369/crude-oil-price-history-chart
2. BASILIO, L.P.; et al. Synergy in using rigid pipelines with subsea production manifolds. Rio Pipeline Conference & Exposition 2015. September 2015.
3. PMI: Project Management Institute. http://www.pmi.org.
4. IPA: Independent Project Analysis. http://www.ipaglobal.com.
5. PMI: PMBOK: A Guide to the Project Management Body of Knowledge. Fifth Edition. 2013.
6. BARBOSA, P. T.; PINHEIRO, N. P. M.; SANTOS JUNIOR, W. L. S. Metodologia FEL: sua importância na avaliação de riscos e redução de impactos em escopo, tempo e custo de projetos complexos de engenharia. XXXIII ENCONTRO NACIONAL DE ENGENHARIA DE PRODUÇÃO. Out-2013.
7. AACE International. Recommended Practice No. 18R-97. Cost estimate classification system: as applied in engineering, procurement, and construction for the process industries. November 2011.
8. BEHNIA, M.; Most accurate two-phase pressure-drop correlation identified. Oil & Gas Journal. International Petroleum News and Technology. September 1991.
9. Revista Petro & Química. Tecnologia a fundo. Seção Petróleo e Gás da Revista Petro & Química. Edição 358, 2014.
10. HOWELL, A; SZATNY, M.; TORRENS, R. From reservoir through process, from today to tomorrow: the integrated asset model. SPE Intelligent Energy Conference & Exhibition. April, 2006.